Vocabulary
Coriolis effect
downwelling
gyre
high tide
longshore current
low tide
neap tide
rip current
spring tide
storm surge
surface current
thermohaline circulation
tidal range
tide
upwelling
wave
Introduction
Ocean water is constantly in motion: north-south, east-west, alongshore, and vertically. Seawater motions are the result of waves, tides, and currents (Figure below). Ocean movements are the consequence of many separate factors: wind, tides, Coriolis effect, water density differences, and the shape of the ocean basins. Water movements and their causes will be discussed in this lesson.
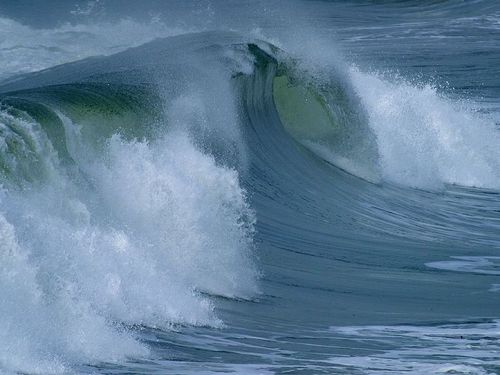
[Figure 1]
Ocean waves transfer energy through the water over great distances.
Waves
Waves have been discussed in previous chapters in several contexts: seismic waves traveling through the planet, sound waves traveling through seawater, and ocean waves eroding beaches. Waves transfer energy and the size of a wave and the distance it travels depends on the amount of energy that it carries.
Wind Waves
This lesson studies the most familiar waves, those on the ocean's surface. Ocean waves originate from wind blowing – steady winds or high storm winds - over the water. Sometimes these winds are far from where the ocean waves are seen. What factors create the largest ocean waves?
The largest wind waves form when the wind
is very strong
blows steadily for a long time
blows over a long distance
The wind could be strong, but if it gusts for just a short time, large waves won’t form.
Wind blowing across the water transfers energy to that water. The energy first creates tiny ripples that create an uneven surface for the wind to catch so that it may create larger waves. These waves travel across the ocean out of the area where the wind is blowing.
Remember that a wave is a transfer of energy. Do you think the same molecules of water that starts out in a wave in the middle of the ocean later arrive at the shore?
Water molecules in waves make circles or ellipses (Figure below). Energy transfers between molecules but the molecules themselves mostly bob up and down in place
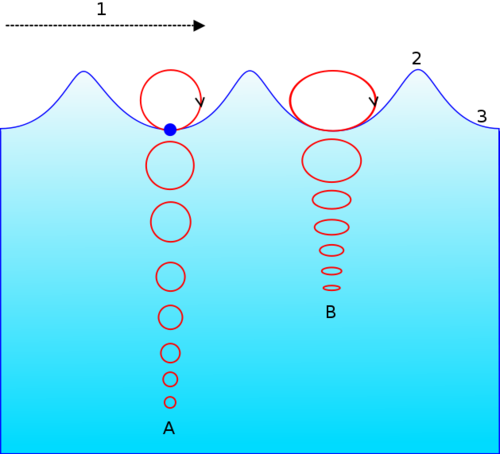
[Figure 2]
The circles show the motion of a water molecule in a wind wave. Wave energy is greatest at the surface and decreases with depth. A shows that a water molecule travels in a circular motion in deep water. B shows that molecules in shallow water travel in an elliptical path because of the ocean bottom.
When does a wave break? Do waves only break when they reach shore? Waves break when they become too tall to be supported by their base. This can happen at sea but happens predictably as a wave moves up a shore. The energy at the bottom of the wave is lost by friction with the ground so that the bottom of the wave slows down but the top of the wave continues at the same speed. The crest falls over and crashes down.
Some of the damage done by storms is from storm surge. Water piles up at a shoreline as storm winds push waves into the coast. Storm surge may raise sea level as much as 7.5 m (25 ft), which can be devastating in a shallow land area when winds, waves, and rain are intense.
A wild video of “Storm Surge” can be seen on National Geographic Videos, Environment Video, Natural Disasters, Landslides,
KQED: Science of Big Waves
Maverick waves are massive. Learning how they are generated can tell scientists a great deal about how the ocean creates waves and especially large waves. Learn more in the video below:
Tsunami
Tsunami are described in the Earthquakes chapter as damaging waves that result from the sharp jolt to the water from an undersea earthquake. Landslides, meteorite impacts, or any other jolt to ocean water may form a tsunami (Figure below). Tsunami can travel at speeds of 800 kilometers per hour (500 miles per hour).
Tsunami have small wave heights and long wavelengths so they are usually unnoticed at sea. As the wave rides up the continental shelf the wave height increases.
A video explanation of tsunami is here: http://www.youtube.com/watch?v=StdqGoezNrY
The wave speed of a tsunami is also slowed by friction with the shallower ocean floor, which causes the wavelength to decrease, creating a much taller wave.
Many people caught in a tsunami have no warning of its approach. Since the wavelength is long, a long time can pass between crests or troughs onshore. In 1755 in Lisbon, an offshore earthquake caused a great deal of damage on land. People rushed to the open space of the shore and discovered that the water was flowing seaward fast. The trough of the tsunami wave reached shore first. People who went out onto the open beach were drowned when the crest of the wave reached shore.
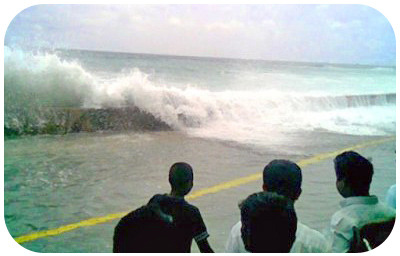
[Figure 3]
A wave from the 2004 Boxing Day Tsunami hits the Maldives in the Indian Ocean.
KQED: Science on the SPOT: Watching the Tides
Large tsunami in the Indian Ocean and more recently Japan have killed hundreds of thousands of people in recent years. The west coast is vulnerable to tsunami since it sits on the Pacific Ring of Fire. Scientists are trying to learn everything they can about predicting tsunamis before a massive one strikes a little closer to home. Learn more in the video below:
Tides
Tides are the daily rise and fall of sea level at any given place. The pull of the Moon’s gravity on Earth is the primarily cause of tides and the pull of the Sun’s gravity on Earth is the secondary cause (Figure below). The Moon has a greater effect because, although it is much smaller than the Sun, it is much closer. The Moon’s pull is about twice that of the Sun’s.
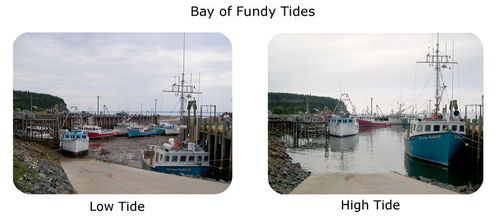
[Figure 4]
High tide (left) and low tide (right) at Bay of Fundy on the Gulf of Maine. The Bay of Fundy has the greatest tidal ranges on Earth at 38.4 feet.
Daily Tide Patterns
To understand the tides it is easiest to start with the effect of the Moon on Earth. As the Moon revolves around our planet, its gravity pulls Earth toward it. The lithosphere is unable to move much but the water is pulled by the gravity and a bulge is created. This bulge is the high tide beneath the Moon. The Moon's gravity then pulls the Earth toward it, leaving the water on the opposite side of the planet behind. This creates a second high tide bulge on the opposite side of Earth from the Moon. These two water bulges on opposite sides of the Earth aligned with the Moon are the high tides.
Since so much water is pulled into the two high tides, low tides form between the two high tides (Figure below). As the Earth rotates beneath the Moon, a single spot will experience two high tides and two low tides every day.
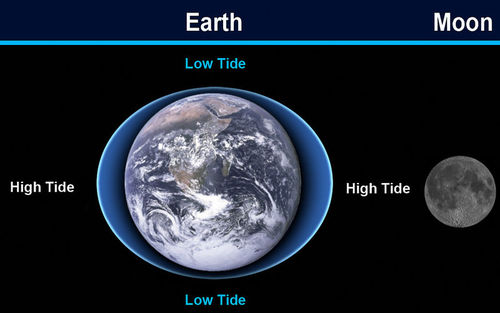
[Figure 5]
The gravitational attraction of the Moon to ocean water creates the high and low tide
The tidal range is the difference between the ocean level at high tide and the ocean at low tide (Figure below). The tidal range in a location depends on a number of factors, including the slope of the seafloor. Water appears to move a greater distance on a gentle slope than on a steep slope.
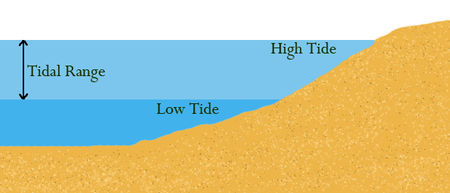
[Figure 6]
The tidal range is the difference between the ocean level at high tide and low tide.
Monthly Tide Patterns
If you look at the diagram of high and low tides in the Figure above, you’ll see that tides are waves. So when the Sun and Moon are aligned, what do you expect the tides to look like?
Waves are additive so when the gravitational pull of both bodies is in the same direction the high tides add and the low tides add (Figure below). Highs are higher and lows are lower than at other times through the month. These more extreme tides, with a greater tidal range, are called spring tides. Spring tides don't just occur in the spring; they occur whenever the Moon is in a new-moon or full-moon phase, about every 14 days.
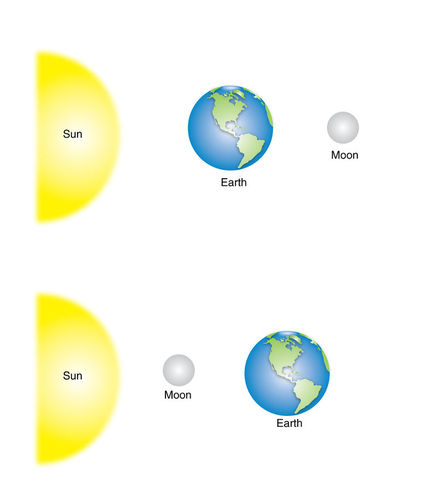
[Figure 7]
Spring tides occur when the tidal bulges from the Moon and Sun are aligned. The Moon is full in this image; in the bottom image the Moon would appear as a new moon.
Neap tides are tides that have the smallest tidal range, and they occur when the Earth, the Moon, and the Sun form a 90o angle (Figure below). They occur exactly halfway between the spring tides, when the Moon is at first or last quarter. How do the tides add up to create neap tides? The Moon's high tide occurs in the same place as the Sun's low tide and the Moon's low tide in the same place as the Sun's high tide. At neap tides, the tidal range relatively small.
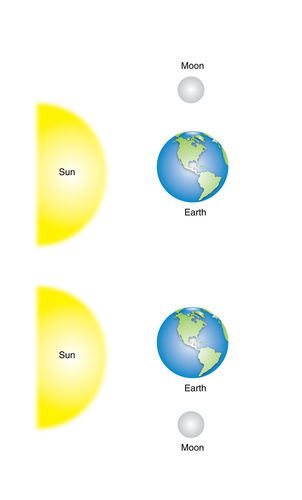
[Figure 8]
Neap tides occur when the Earth, the Sun, and the Moon form a right angle; the Moon is in its first or third quarter.
High tides occur about twice a day, about every 12 hours and 25 minutes. The reason is that the Moon takes 24 hours and 50 minutes to rotate once around the Earth so the Moon is over the same location 24 hours and 50 minutes later. Since high tides occur twice a day, one arrives each 12 hours and 25 minutes. What is the time between a high tide and the next low tide?
Some coastal areas do not follow this pattern at all. These coastal areas may have one high and one low tide per day or a different amount of time between two high tides. These differences are often because of local conditions, such as the shape of the coastline that the tide is entering.
Surface Currents
Ocean water moves in predictable ways along the ocean surface. Surface currents can flow for thousands of kilometers and can reach depths of hundreds of meters. These surface currents do not depend on weather; they remain unchanged even in large storms because they depend on factors that do not change.
Surface currents are created by three things:
global wind patterns
the rotation of the Earth
the shape of the ocean basins
Surface currents are extremely important because they distribute heat around the planet and are a major factor influencing climate around the globe.
Global Wind Patterns
Winds on Earth are either global or local. Global winds blow in the same directions all the time and are related to the unequal heating of Earth by the Sun -- that is, more solar radiation strikes the equator than the polar regions –- and the rotation of the Earth -- that is, the Coriolis effect. The causes of the global wind patterns will be described in detail in the Earth's Atmosphere chapter.
Water in the surface currents is pushed in the direction of the major wind belts:
trade winds: east to west between the equator and 30oN and 30oS
westerlies: west to east in the middle latitudes
polar easterlies: east to west between 50o and 60o north and south of the equator and the north and south pole
Earth’s Rotation
Wind is not the only factor that affects ocean currents. The Coriolis effect describes how Earth’s rotation steers winds and surface ocean currents (Figure below). Coriolis causes freely moving objects to appear to move to the right in the Northern Hemisphere and to the left in the Southern Hemisphere. The objects themselves are actually moving straight, but the Earth is rotating beneath them, so they seem to bend or curve.
An example might make the Coriolis effect easier to visualize. If an airplane flies 500 miles due north, it will not arrive at the city that was due north of it when it began its journey. Over the time it takes for the airplane to fly 500 miles, that city moved, along with the Earth it sits on. The airplane will therefore arrive at a city to the west of the original city (in the Northern Hemisphere), unless the pilot has compensated for the change. So to reach his intended destination, the pilot must also veer right while flying north.
As wind or an ocean current moves, the Earth spins underneath it. As a result, an object moving north or south along the Earth will appear to move in a curve, instead of in a straight line. Wind or water that travels toward the poles from the equator is deflected to the east, while wind or water that travels toward the equator from the poles gets bent to the west. The Coriolis effect bends the direction of surface currents to the right in the Northern Hemisphere and left in the Southern Hemisphere.
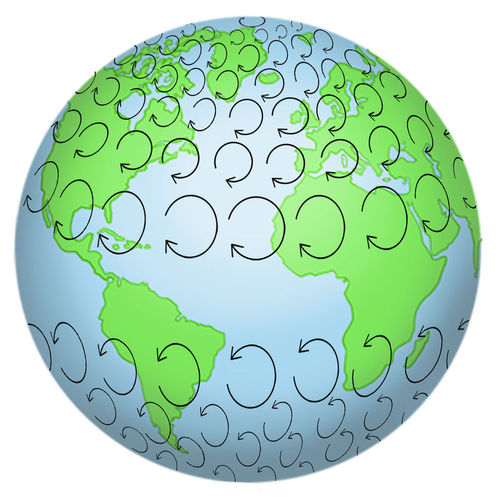
[Figure 9]
The Coriolis effect causes winds and currents to form circular patterns. The direction that they spin depends on the hemisphere that they are in.
Coriolis effect is demonstrated using a metal ball and a rotating plate in this video. The ball moves in a circular path just like a freely moving particle of gas or liquid moves on the rotating Earth (5b)
Shape of the Ocean Basins
When a surface current collides with land, the current must change direction. In the Figure below, the Atlantic South Equatorial Current travels westward along the equator until it reaches South America. At Brazil, some of it goes north and some goes south. Because of Coriolis effect, the water goes right in the Northern Hemisphere and left in the Southern Hemisphere.
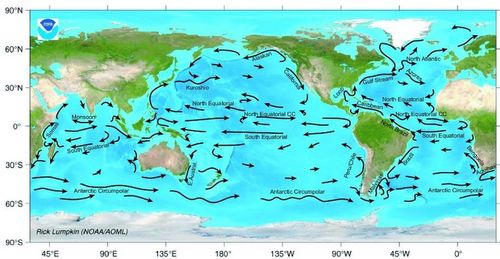
[Figure 10]
The major surface ocean currents.
You can see on the map of the major surface ocean currents that the surface ocean currents create loops called gyres(Figure below). The Antarctic Circumpolar Current is unique because it travels uninhibited around the globe. Why is it the only current to go all the way around?
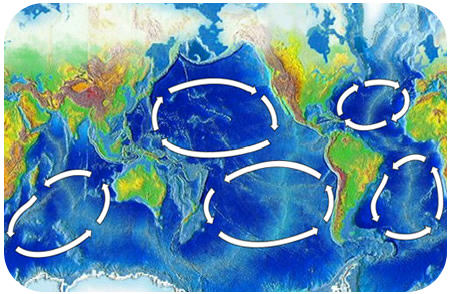
[Figure 11]
The ocean gyres. Why do the Northern Hemisphere gyres rotate clockwise and the Southern Hemisphere gyres rotate counterclockwise?
Local Surface Currents
The surface currents described above are all large and unchanging. Local surface currents are also found along shorelines (Figure below). Two are longshore currents and rip currents.
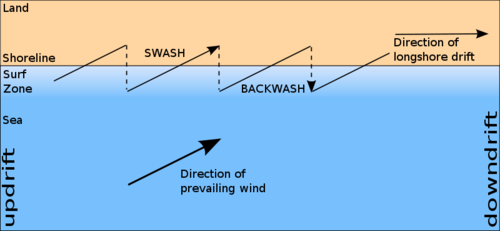
[Figure 12]
Longshore currents move water and sediment parallel to the shore in the direction of the prevailing local winds.
Rip currents are potentially dangerous currents that carry large amounts of water offshore quickly. Each summer in the United States at least a few people die when they are caught in rip currents.
Effect on Global Climate
Surface currents play an enormous role in Earth’s climate. Even though the equator and poles have very different climates, these regions would have more extremely different climates if ocean currents did not transfer heat from the equatorial regions to the higher latitudes.
The Gulf Stream is a river of warm water in the Atlantic Ocean, about 160 kilometers wide and about a kilometer deep. Water that enters the Gulf Stream is heated as it travels along the equator. The warm water then flows up the east coast of North America and across the Atlantic Ocean to Europe (Figure below). The energy the Gulf Stream transfers is enormous: more than 100 times the world's energy demand.
The Gulf Stream's warm waters raise temperatures in the North Sea, which raises the air temperatures over land between 3 to 6oC (5 to 11oF). London, U.K., for example, is at the same latitude as Quebec, Canada. However, London’s average January temperature is 3.8oC (38oF), while Quebec’s is only -12oC (10oF). Because air traveling over the warm water in the Gulf Stream picks up a lot of water, London gets a lot of rain. In contrast, Quebec is much drier and receives its precipitation as snow.
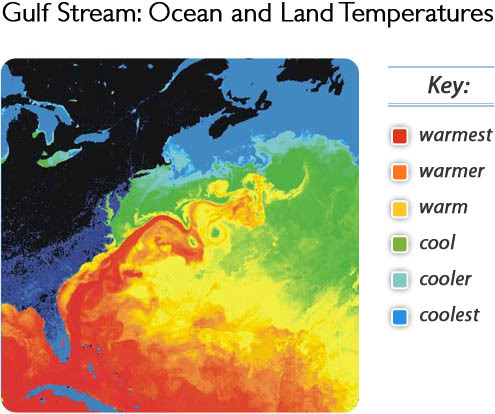
[Figure 13]
In a satellite image of water temperature in the western Atlantic it is easy to pick out the Gulf Stream, which brings warmer waters from the equator up eastern North America.
Deep Currents
Thermohaline circulation drives deep ocean circulation. Thermo means heat and haline refers to salinity. Differences in temperature and in salinity change the density of seawater. So thermohaline circulation is the result of density differences in water masses because of their different temperature and salinity.
What is the temperature and salinity of very dense water? Lower temperature and higher salinity yield the densest water. When a volume of water is cooled, the molecules move less vigorously so same number of molecules takes up less space and the water is denser. If salt is added to a volume of water, there are more molecules in the same volume so the water is denser.
Changes in temperature and salinity of seawater take place at the surface. Water becomes dense near the poles. Cold polar air cools the water and lowers its temperature, increasing its salinity. Fresh water freezes out of seawater to become sea ice, which also increases the salinity of the remaining water. This very cold, very saline water is very dense and sinks. This sinking is called downwelling.
This video lecture discusses the vertical distribution of life in the oceans. Seawater density creates currents, which provide different habitats for different creatures (5d):
Two things then happen. The dense water pushes deeper water out of its way and that water moves along the bottom of the ocean. This deep water mixes with less dense water as it flows. Surface currents move water into the space vacated at the surface where the dense water sank (Figure below). Water also sinks into the deep ocean off of Antarctica.
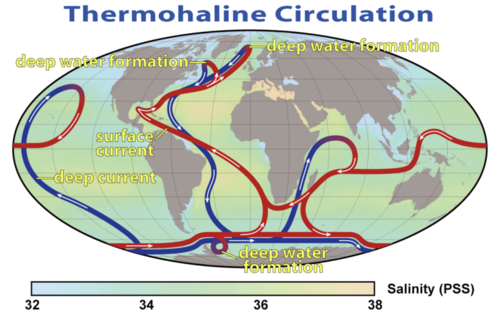
[Figure 14]
Cold water (blue lines) sinks in the North Atlantic, flows along the bottom of the ocean and upwells in the Pacific or Indian. The water then travels in surface currents (red lines) back to the North Atlantic. Deep water also forms off of Antarctica.
Since unlimited amounts of water cannot sink to the bottom of the ocean, water must rise from the deep ocean to the surface somewhere. This process is called upwelling (Figure below).
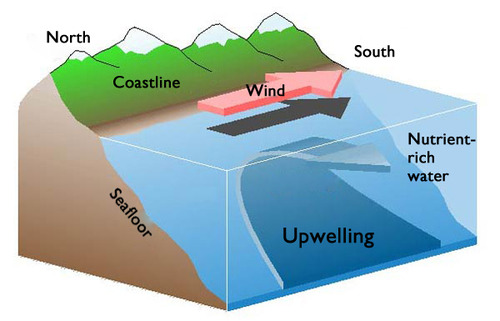
[Figure 15]
Upwelling forces denser water from below to take the place of less dense water at the surface that is pushed away by the wind.
Generally, upwelling occurs along the coast when wind blows water strongly away from the shore. This leaves a void that is filled by deep water that rises to the surface.
Upwelling is extremely important where it occurs. During its time on the bottom, the cold deep water has collected nutrients that have fallen down through the water column. Upwelling brings those nutrients to the surface. Those nutrient support the growth of plankton and form the base of a rich ecosystem. California, South America, South Africa, and the Arabian Sea all benefit from offshore upwellin
Upwelling also takes place along the equator between the North and South Equatorial Currents. Winds blow the surface water north and south of the equator so deep water undergoes upwelling. The nutrients rise to the surface and support a great deal of life in the equatorial oceans.
Lesson Summary
Ocean waves are energy traveling through the water.
Most ocean waves are generated by wind. Tsunami are exceptionally long wavelength waves usually caused by earthquakes.
Tides are produced by the gravitational pull of the Moon and Sun.
Spring tides have large tidal ranges and occur at full and new moons, when Earth, Moon, and Sun are all aligned.
Neap tides have low tidal ranges and occur at first and last quarter moons, when the Moon is at right angles to the Sun.
Ocean surface currents are produced by global winds, the Coriolis effect and the shape of each ocean basin.
The Pacific and Atlantic Oceans have a circular pattern of surface currents called gyres that circle clockwise in the Northern Hemisphere and counterclockwise in the Southern. The Indian Ocean only has a counterclockwise gyre.
Surface ocean circulation brings warm equatorial waters towards the poles and cooler polar water towards the equator.
Thermohaline circulation drives deep ocean currents.
Upwelling of cold, nutrient-rich waters creates biologically rich areas where surface waters are blown away from a shore, or where equatorial waters are blow outward.